| Net treatment | Processes of the snowfluent treatment | Atomization/crystallization | Aging of the snowpack | Runoff and residue | Bioaerosols
Net Treatment
Appendix B contains constituent concentrations and loads for all the parameters measured. Table 1 gives a summary of the some of the more critical compounds examined.
Table 1. Typical Concentration Reductions During the Snowfluent Process.
Parameter | Raw Manure(n = 3) | Fresh Snow (n = 5) | Runoff (n > 6000) | Net Reduction |
Mean Concentration | ±SE | Mean Concentration | ±SE | Mean Concentration | ±SE |
Biochemical Oxygen Demand (BOD) (mg/L) | 76524 | 76 | 6925 | 49 | 3360 | 55 | 48% |
Total Kjeldahl Nitrogen (TKN) (mg/L) | 2431 | 240 | 2462 | 126 | 946 | 15 | 61% |
Inorganic Nitrogen (NO2+NO3) (mg/L) | 1.244 | 0.173 | 0.866 | 0.135 | 0.141 | 0.002 | 89% |
Total Phosphorous (TP) (mg/L) | 424.5 | 43.7 | 329.3 | 32.1 | 131.0 | 1.6 | 69% |
Faecal Coliforms (FC) (CFU/100mL) | 6.71x106 | 5.61x105 | 4.63x105 | 3.34x105 | 27 | <1 | 5-log (99.999%) |
Equivalent Water Content (L) | 212,906 | 91,000 | 82,000 |  |
Based on their previous work with Snowfluent, Delta Engineering predicted a 20-30% loss of water volume over the entire Snowfluent process. This loss represents evaporation of the water as it is atomized within the snowgun, and sublimation of the ice crystals as they fall to the ground, and mature on the snowpack. With this trial a water volume loss on the order of approximately 60% was observed, but this number is likely an overestimate. It was estimated that 75% of the snow in the immediate area was actually within the borders of the test plot, and would be collected as the plot melted and drained. 75% is probably an over estimate, since it addresses only the snow lying directly outside the borders of the test plot, and doesn't consider the fine snow that was deposited farther away.
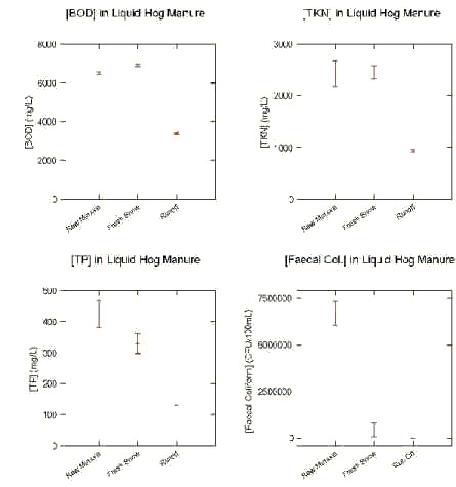
Figure 1. Mean Concentrations for BOD, TKN, TP, and Faecal Coliforms During the Snowfluent Process.
The large loss of water volume in the Atomization/Crystallization (A/C) phase of the process resulted in no significant decrease, in some cases even an increase, in most constituent concentrations between the raw manure and the fresh snow (Figure 1). This concentration effect is believed to be the result of the A/C process losing proportionately more water vapour than constituent. This effect was not observed with malting plant effluent trials performed under similar climatic conditions (Wuite et al, 1997), possibly due to the difference in scale and nature of the various constituent concentrations between malting wastewater and hog manure.
Generally speaking, there was a reduction in all constituent concentrations between the raw manure and runoff phases. When the biochemical oxygen demand (BOD) concentration of the initial liquid manure is compared to the BOD concentration of the runoff volume, a 48% reduction is observed (Table 1). The Total Kjeldahl Nitrogen (TKN) concentration is reduced by 61%, the sum of nitrate and nitrite loads are reduced by 89%, and the Total Phosphorous (TP) concentration is reduced by 69%.
Total Coliform (TC) counts in the various stages of the Snowfluent process were higher than the Faecal Coliform (FC) counts, and did not drop significantly with treatment (Appendix B).The staff of the microbiology lab at Alberta Research Council (ARC) Vegreville speculate that this is more likely a result of the lab analysis methodology and the highly nutritive wastewater, and not necessarily an accurate reflection of what was in the water. The TC plates grew many colonies of 'background' organisms such as other types of bacteria and fungi, which interfered with the TC counts. The Faecal Coliform levels were more consistent with their experience of liquid manure.
For a number of the runoff samples, the concentration of NH3 was higher than that measured for TKN. Since TKN is a sum of organic nitrogen and inorganic nitrogen, including NH3, this is suspect. The water chemistry lab at ARC retested these samples, and confirmed the readings, but could not offer any definitive explanation..
During snowmaking, there were significant levels of odour directly within the snowplume. Outside the snowfall area, odours were considerably less. At a distance of 30 m from the plume, odours were minimal. Observations of odour levels at distances greater than 30 m downwind were not made. The freshly made snowpack could be smelled when standing directly beside it. At a distance of 10 m the snowpack odour could not be distinguished from the barns or ice-covered lagoon. The surface odour of the snowpack continually decreased over the winter as the snowpack aged. However, when the deeper layers of the snowpack were exposed, a distinct 'ammonia odour' was observed. The meltwater was grey/brown in colour, and had a definite 'manure' odour to it. However the odour of the meltwater was considerably less than that of untreated liquid manure. The residue left on the liner was grey and ash textured with a faint manure odour which decreased as it dried.
Most of the nitrogen load in both the raw manure and the Snowfluent meltwater, was in the form of ammonia. The volatile nature of ammonia is likely the reason for most of the nitrogen loss associated with liquid hog manure management. Nitrogen loss and retention is discussed further in section 3.0, Nutrient Retention.
Processes of the Snowfluent Treatment
The Snowfluent process can be thought of as a series of three sub-processes. Atomization/crystallization (A/C) occurs as the raw wastewater is divided into small (approx. 200 µm in diameter) droplets and blown into the air where it freezes into ice crystals. Snowpack aging occurs as the snowpack is exposed to diurnal and seasonal fluctuations in many climatic parameters such as temperature, humidity, and solar radiation. These fluctuations cause physical and chemical changes within the snowpack. Melting is the separation of the snowpack into liquid runoff and a solid residue.
Atomization/Crystallization
By mixing the raw manure with high pressure air within the snowgun, not only is the stream of wastewater atomized, but the expansion of the compressed air as it exits the snowgun absorbs heat, lending additional cooling to the spray.
The treatment effect of the A/C phase was dependant on volatilization and precipitation. Coliform concentrations were also reduced via quick freezing and UV exposure, discussed later in this section. Volatilization occurred when compounds such as CO2, ammonia, and some volatile organics were released as a vapour from the atomized droplets. Constituent decreases attributed to volatilization can be explained by the nature of atomized water droplets. When a volume of water resides in a lagoon, processes such as the chemical oxidation of oxidizable compounds, and release of soluble ammonia to a gaseous form are restricted by the amount of these compounds that have access to the air. This depends on the available surface area, and the number of the molecules which are close enough to the air/liquid interface to be affected. It is estimated that when a 1 m3 volume of water is atomized through the Snowfluent nozzle to droplets in the order of 200 µm in diameter, its surface area is increased approximately 30,000 times. Also, due to the minute volume of water contained in each drop, no molecule within the droplet is greater than 100 µm from the surface, where oxygen can diffuse into the liquid, and volatile compounds can diffuse out.
Precipitation occurred when the action of freezing encouraged dissolved compounds to form insoluble or slightly soluble compounds (Huber and Palmateer, 1985). The loss of acidic compounds such as CO2 caused a rise in the pH (approx. 0.79 pH units in this test) of the water as it moved from the raw effluent phase to the fresh snow phase of the Snowfluent process, possibly catalysing further precipitation. These insoluble or slightly soluble precipitates, initially trapped in the matrix of the ice crystals, separated physically from the water when snowmelt began.
The A/C phase of the Snowfluent process also produced the greatest reductions in faecal pathogens. This was due to the nature of how atomized water freezes. Small droplets (approx 200 microns in diameter) of atomized water have less latent heat energy than large volumes of water, and also have a larger surface area to volume ratio. Small droplets freeze rapidly with a greater proportion of their volume exposed to the cold air at the surface. Rapid freezing of bacterial cells causes rupturing of the cell membrane by expanding ice crystals.
Fedorak and Westlake (1980) observed that bioaerosols (and by extension, bacteria suspended in airborne water/ice droplets) show a higher kill rate during the day than during the night due to exposure to the ultraviolet (UV) component of natural sunlight. The efficiency of this UV disinfection process also depends on how long the atomized droplets remain in air and exposed to sunlight, before falling to the ground and becoming covered by overlying layers of snow. For this pilot test the snowmaking crew had to operate at night and thus UV exposure would not be a significant factor in the observed reductions of pathogens at snowmaking.
Both of these effects, membrane disruption and UV exposure, can be enhanced by performing the snowmaking operation during optimum conditions of temperature, humidity, and daylight. The colder and drier the air is, the faster the membrane rupturing ice-crystals form, and the finer the crystal sizes are, prolonging their suspension in the plume and exposure to light.
Aging of the Snowpack
As the snowpack sat exposed to diurnal and seasonal changes in its environment, there were additional losses of volatile compounds, separation of the meltwater from precipitates, and prolonged exposure of pathogens to UV light.
BOD underwent large reductions during the aging process. Larger particles of organic matter were filtered out as the melt water moved from the top to the bottom of the snow pack. As the pack receded, a layer of organic particles was left on top of the snowpack. This residue was eventually left behind when the snow pack melted.
While the surface odour of the snowpack continually decreased as it aged, the lower depths of the snowpack released a strong ammonia smell when exposed during snowpack sampling. This ammonia smell was not apparent in the snow at the surface of the snowpack. This would suggest that as the snowpack recedes exposing deeper layers of snow, ammonia is slowly released to the atmosphere from the surface layers of the snowpack.
Runoff and Residue
After being processed in the A/C and aging phases, the remaining snow pack and its associated loads were contained in two final products, runoff and residue. The location for the test plot in this study was deliberately selected for its steep slope, in order to direct all runoff toward a measurement/collection area. This allowed a significant portion of the insoluble or slightly soluble precipitates separated from the meltwater during the Snowfluent process, to be washed out with the runoff. In the absence of a steep slope, a greater portion of these solids should remain with the residue component, allowing a more efficient separation of clean liquid from laden residue. To keep the separated solid and liquid fractions from recombining through resuspension in the runoff would require careful site selection.
During the initial stages of melt in the snowpack a 'flushing front' was formed. At the start of the melt season, the top of the pack was exposed first to melting conditions. Since the melt water forms at the top of the pack it must move down, via gravity, through the remaining snow mass to exit at the bottom. As this wet front migrated down through the pack, it picked up soluble compounds and small insoluble particles, before exiting at the snow/soil interface. Johannessen and Henriksen (1978) observed that 41-80% of the chemical load of a natural snowpack is released with the first 30% of the runoff volume. The Percentage of Total Runoff illustrated in (Figure 2) shows that the rate of discharge from the lined plot was reasonably consistent throughout the melt period. Despite this consistent rate of melt, the release of the snowpack's chemical loads was quite heavily weighted towards the beginning of the melt period (Figure 3). The Snowfluent pack made from liquid hog manure shed 55-80% of its chemical loads in the first 30% of the meltwater volume. For a production scale application, this might mean that the initial concentrated runoff be returned to the primary lagoon, and that the cleaner, second phase of runoff be recycled to a holding pond for flushing of the barn.
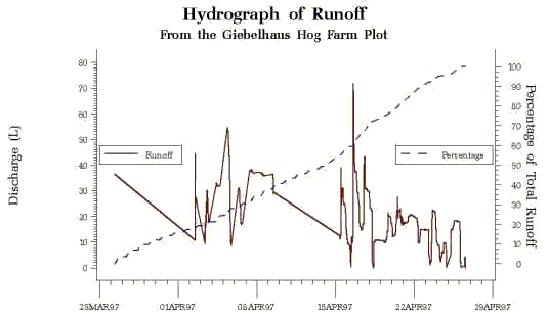
Figure 2. Hydrograph of Runoff from the Giebelhaus Hog Farm Test Plot
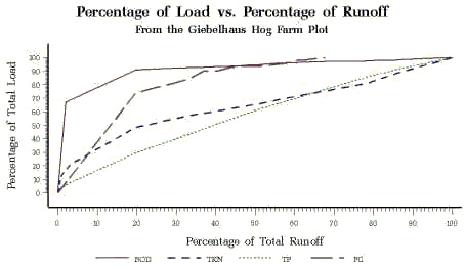
Figure 3. The Flushing of BOD, TKN, TP, and Faecal Coliform Loads from the Snowpack as Runoff
Bioaerosols
Handling wastewater represents an inherent risk to the operator. Wastewaters, either municipal or agricultural, contain many microorganisms, among other contaminants, that can affect human health. Health risks associated with aerosolized microorganisms include eye and nose irritation, cough with phlegm, wheezing, attacks of breathlessness, headache, dizziness, tiredness, nausea and Organic Dust Toxic Syndrom (ODTS) (Melbostad et al. 1994; Van der Werf 1996). The process of freeze crystallization and atomization of raw wastewater with the Snowfluent technology allows bacteria to become airborne. Viable aerosolized bacteria can potentially affect human respiratory health. Therefore, it was essential to determine whether this represents a significant health concern to the Snowfluent operator.
Bioaerosol concentrations from Snowfluent were dependent on the distance from the snowgun along the trajectory path of the Snowfluent plume. The total number of bacteria (Heterotrophic Plate Count (HPC)) upwind of the snowmaking guns yielded relatively low background levels (Table 2). In contrast, bioaerosol samples collected immediately downwind of the snowmaking guns and directly in the snow plume resulted in high HPC, Faecal Coliform and Faecal enterococci levels. At approximately 100 m downwind of the snowmaking operation, all bioaerosols were comparable to upwind sample concentrations.
Table 2. Bioaerosols from Snowfluent Made of Liquid Hog Manure.
Approximate distance
downwind of snow gun
(m) | n | Heterotrophic Plate Count (CFU/100 L air) | Faecal Coliform
(CFU/ 100 L air) | Faecal Enterococcus
(CFU/100 L air) |
upwind | 2 | 110 | 14 | 10 |
6 | 2 | 29 010 | 2 403 | 1 007 |
16 | 2 | 205 000 | 8 600 | 3 250 |
100 | 2 | 130 | 13 | 13 |
There are no bioaerosol data to compare the Snowfluent technology with other liquid waste treatment technologies. Although no guidelines exist in Alberta or Canada for occupational exposure to aerosolized microorganisms, Donham (1989) proposed exposure thresholds of 63,000 CFU/100 L air for total bacteria in Swedish swine buildings where workers experienced a significant decrease in pulmonary function tests. Only the total bacteria levels observed directly in the plume (16 m downwind) exceeded these proposed limits.
The bacteria levels found immediately downwind of the snowmaking guns (approximately 6 m) were similar to bacteria levels found in swine confinement buildings. For example, Butera et al. (1991), Donham (1989) and Elliott et al. (1976) found total bacteria levels in swine facilities to average 46 200, 40 000, and 23 500 CFU/100 L of air, respectively. Only the levels of viable HPC bacteria found directly in the plume exceeded levels found in swine facilities. Furthermore, Elliott et al (1976) found that faecal coliforms only comprised a small percentage (<7%) of the total aerosolized bacteria. Similar results were found with the Snowfluent technology.
Apart from when they are required to work directly within the plume, Snowfluent operators are not expected to be exposed to bioaerosol levels higher than what they would encounter inside a typical hog barn. When the nature of their duties does require them to work within the plume they are exposed to bioaerosol levels that are higher than the safety limits proposed by Donham (1989). For this reason it is recommended that Snowfluent operators who are required to spend extended periods of time in the plume, wear a protective breathing apparatus to avoid any increase in their risk of associated respiratory health problems. |
|