| Problem | Literature review | Study description | Major findings | Applied questions | References
Problem
Fall to spring soil structure changes can lead to wind erosion in southern Alberta. Soil characteristics such as aggregate size distribution and surface roughness can be used to assess soil structural changes over time. To accurately predict these changes, soil surface samples need to be intensively measured. Knowing the exact time that aggregate size distributions and surface roughness would be conducive to wind erosion would be beneficial for implementing wind erosion prevention and control measures for specific sites and climates. A study was initiated at the Lethbridge Research Centre to quantify overwinter dry aggregate size distribution and surface roughness changes at discrete intervals between fall and spring on conventionally (i.e. cultivated) fallowed fields.
Literature Review
Wind erosion is still a contributing factor to soil degradation in the Canadian Prairies and especially in southern Alberta11. One soil property that can influence the wind erosion potential is the dry aggregate size distribution which is determined by sieving dry soil in a rotary sieve12,4. This allows determination of the soil erodible fraction, which has been defined as the percent of soil aggregates less than 0.84 mm in diameter3. Generally, soils with an erodible fraction greater than 60% are considered at high erosion risk1. Erosion risk also depends on aggregate density and wind speeds.
Surface roughness can also determine the erosion potential of fields. As fields become smoother, the potential wind speed at the soil surface becomes greater. Surface roughness is due to clods (random roughness) and tillage ridges (oriented roughness). Larger clods and tillage ridges perpendicular to the prevailing wind direction are more effective for wind erosion control2,5.
The soil erodible fraction changes overwinter and has been known to increase wind erosion risk, especially after cultivated fallow, a common practice for water conservation in dryland
cropping systems on the semi-arid Canadian Prairies7. The erodible fraction has been measured in fall and again in spring for small grain-fallow rotations of various fallow management techniques8. However, with the advent of a new computer model known as the Wind Erosion Prediction System (WEPS) which is an erosion predictor and quantifier based on a daily-time-step process, there is a need to monitor changes of the erodible fraction between fall and spring6.
Study Description
For the winters of 1992-93 (Fairfield site), 1993-94 (Wilson site), and 1994-95 (Lethbridge site), changes in the erodible fraction were monitored after a fallow season in a dryland small grain management system near Lethbridge, Alberta. All surface soils were clay loam texture with approximately 1.7% organic carbon. The studies began immediately after a final fall tillage operation. Soil surface (0-2.5 cm depth) aggregate size distribution samples and surface roughness were taken periodically and only when the surface was bare of snow. Surface roughness was measured at the Wilson (1993-94) and Lethbridge (1994-95) sites using the chain surface roughness method10. Roughness was recorded as the centimetres less than the straight length of the chain. The larger the number, the greater the roughness.
The Fairfield site had barley silage removed for three consecutive years prior to the fallow season preceding the overwinter study. During the fallow season in the summer of 1992, the Fairfield site had only one pass of a blade cultivator and was chemical fallowed. In August, 1992 a hailstorm pulverized the remaining residue and after a pass with a cultivator to initiate the study, there was virtually no surface residue.
The Wilson site had been in a no-till system for seven years prior to the fallow season in the summer of 1993, which included four passes of a heavy duty cultivator with rod.
The Lethbridge site was in a long-term wheat-fallow system (40 years) which had been fallowed with a one-way disker.
Major Findings
All sites showed general increases in the erodible fraction from fall to spring (Figures 1a, b, c). Only the Fairfield site exceeded the critical 60% erodible fraction threshold (Figure 1a). The Fairfield site took only 78 days (September 18 to December 4) to increase from 39% to 61% erodible fraction. Using the 60% erodible fraction erosion threshold1, this field would have been prone to erosion for up to five months before spring seeding. However, on February 1, 1993, the erodible fraction decreased due to on-site snow melt and crusting of the soil surface. But within 30 days on March 3, the erodible fraction was once again above 60%. Surface roughness was not measured at this site.
The Wilson site (1993-94) had the lowest erodible fraction (13%) at the onset of the study compared to the other sites and only reached a maximum of 44% erodible fraction (Figure 1b). Wind erosion was monitored on this site and on February 9, 1994 wind erosion began with only 39% erodible fraction and continued, on days with sufficient wind, until spring seeding.
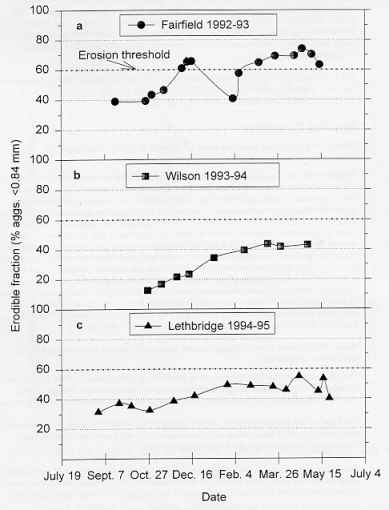
Figure 1. Erodible fraction for three overwinter sites near Lethbridge, Alberta
The surface roughness at the Wilson site generally decreased over the study period and ranged from 15.1 to 3.7 cm (Figure 2a). Only roughness parallel to tillage direction or random roughness (clod size related) is reported since tillage ridges were negligible. The surface roughness at erosion onset on February 9 was between 6.9 and 5.1 cm. It must also be noted that the width of the field was 200 m, about 10 times wider than the other sites. Large fetch distances allow maximum wind velocities to develop over bare fields and increase erosion potential.
The Lethbridge site (Figure 1c) ranged from 31 to 55% erodible fraction for the duration of the study. Random surface roughness also decreased over time and ranged from 14.4 to 3.3 cm (Figure 2b). Erosion was not monitored at this site.
Applied Questions
What field preparation practices could be adopted to reduce overwinter soil degradation by wind erosion?
Producers who rely on soil clods for wind erosion protection must realize that the overwinter soil erodible fraction and surface roughness are dynamic soil characteristics. Under certain soil moisture and climatic conditions the protection from soil clods may dissipate rapidly. All of these study sites had increases in the erodible fraction and an accompanying decrease in surface roughness over the study period. This is one reason why adoption of zero tillage fallow or continuous cropping systems is attractive to producers with fields prone to wind erosion. Standing grain stubble is a definite deterrent to erosion and is not as susceptible to change due to climatic conditions. However, in drought years where crop residue is scant or if residue is lost due to fire, investigators8,9 have found that the erodible fraction on a zero tillage field may be greater than on a conventionally fallowed field. Other alternatives may be to fallow with wide blade cultivators, plant field shelterbelts, and spread manure or straw on the affected area. If these measures are insufficient, it may be necessary to implement emergency measures such as deep rip tillage of clay soils or listing of sandy soils (using lister shovels to create ridges and bring firmer subsoil up to the surface).
What effect do long-term cropping systems have on changes in soil erodible fraction and surface roughness?
This study suggests that long-term cropping systems may affect initial erodible fraction and subsequent overwinter changes and to a lesser extent surface roughness. The sites in this study had quite similar soil texture and organic carbon levels but variable cropping histories.
The Fairfield site had almost all the surface residue removed for three years previous to the study initiation. This management technique resulted in a rapid increase in the erodible fraction and high erosion risk for a majority of the study.
The Wilson site, which had the lowest initial erodible fraction of all sites, had been in a zero tillage system for seven years prior to the fallow season in 1993. Surface residue can protect the soil from climatic elements either directly or by trapping an insulative snow cover, but
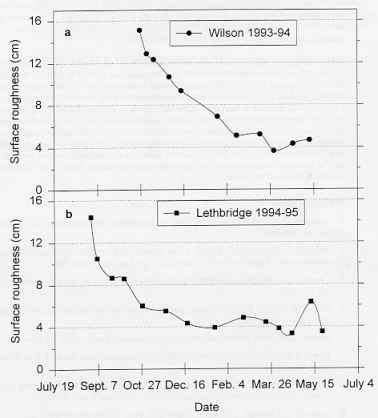
Figure 2. Parallel to tillage direction surface roughness (random) at two overwinter sites near Lethbridge, Alberta
since surface residue amounts were similar to amounts at the other sites at study initiation, some other indirectly related factors resulting from zero tillage management appear to have had some effect on the erodible fraction. Physical disruption from years of tillage may be detrimental to soil structure strength. A zero tillage management system allows a net organic matter buildup near the soil surface because of the lack of tillage disturbance. It was also noted that the soil environment under the zero tillage system at the Wilson site promoted significant worm populations compared with the other sites and associated worm castes have been known to be resistant to disruptive climatic forces. These factors may have contributed to the low erodible fractions measured over the study period even though wind erosion losses were still measured at the site. Other erosion factors such as surface roughness, fetch distances and wind speeds are also obviously important.
Surface roughness ranges were similar for the Wilson (15.1 to 3.7 cm) and Lethbridge sites (14.4 to 3.3 cm) even though the erodible fraction range was from 13 to 44% (31% range) for the Wilson site and 31 to 55% (24% range) for the Lethbridge site. Similar surface roughness changes occurred by the end of the study even though the study duration was longer at the Lethbridge site than the Wilson site. This would appear to indicate that cropping system may not be as closely related to overwinter surface roughness changes as it is to erodible fraction. Perhaps erodible fraction is a more sensitive measurement than chain surface roughness. The erosion at the Wilson site and not at the Lethbridge site may have affected surface roughness in a different manner as well. It also should be noted that the particular weather for each year may be just as or more important than the cropping system history for each site for surface roughness and erodible fraction.
References
- Anderson, C.H. and Wendhardt, A. 1966. Soil erodibility, fall and spring. Can. J. Soil Sci. 46: 255-259.
- Arika, C.L., Gregory, J.M., Borelli, J. and Zartman, R.E. 1986. A ridge and clod wind erosion model. Paper No. 86-2531. Am. Soc. Agr. Eng., St. Joseph, MI.
- Chepil, W.S. 1942. Measurement of wind erosiveness by dry sieving procedure. Sci. Agr. 23: 154-160.
- Chepil, W.S. 1952. Improved rotary sieve for measuring state and stability of dry soil. Soil Sci. Soc. Am. Proc. 18: 13-18.
- Fryrear, D.W. 1984. Soil ridges-clods and wind erosion. Trans. ASAE 18:445-448.
- Hagen, L.J. 1991. A wind erosion prediction system to meet user needs. J. Soil Water Cons. 46: 106-111.
- Larney, F.J., Bullock, M.S., McGinn, S.M. and Fryrear, D.W. 1995. Quantifying wind erosion on summer fallow in southern Alberta. J. Soil Water Cons. 50: 91-95.
- Larney, F.J., Lindwall, C.W. and Bullock, M.S. 1994. Fallow management and overwinter effects on wind erodibility in southern Alberta. Soil Sci. Soc. Am. J. 58:1788-1794.
- Layton, J.B., Skidmore, E.L. and Thompson, C.A. 1993. Winter-associated changes in dry-soil aggregation as influenced by management. Soil Sci. Soc. Am. J. 57: 1568-1572.
- Saleh, A. 1993. Soil roughness measurement: chain method. J. Soil Water Cons. 48: 527-529.
- Wall, G.J., Pringle, E.A., Padbury, G.A., Rees, H.W., Tajek, J., van Vliet, L.J.P., Stushnoff, C.T., Eilers, R.G. and Cossette, J.-M. 1995. Erosion. Pages 61-76 in D.F. Acton and L.J. Gregorich (eds.), The health of our soils: towards sustainable agriculture in Canada. Centre for Land and Biological Resources Research, Agriculture and Agri-Food Canada, Ottawa, ON. Publication 1906/E.
- Zobeck, T.M. 1991. Soil properties affecting wind erosion. J. Soil Water Cons. 46: 112-118.
Return to CAESA Soil Quality Program Research Factsheets
This information is provided by M.S. Bullock and F.J. Larney.
|
|