| Abstract | Introduction | Materials and methods | Results and discussion
Abstract
Since the early 1970's, soil nitrate nitrogen (NO3-N) level has been used as a basis for N fertilizer recommendations in western Canada. However, soil nitrate N does not represent the supplying power of soil N for plant uptake. Furthermore, the nitrate concentration in soil changes depending on the time and ambient conditions at sampling. Researches in the past have been made to use mineralization potential to predict N supply power for plant growth. There are two methods to estimate the potential mineralization N: a laboratory incubation and a chemical extraction method. The laboratory incubation method requires a long time to obtain a measurement. By comparison the chemical extraction method (e.g. hot KCl extraction) provides a quick estimation of potential mineralizable N, but the method requires calibration with laboratory incubation results.
Alberta Agriculture, Food and Rural Development has established benchmark sites to monitor soil property changes from agricultural practices. Hot KCl extractable N and soil organic matter were determined each year in these sites. Using the results from the benchmark sites, we developed an approach to estimate Nt from soil organic matter based on an equation of Nt = No(1-e-kt)y, and validated the calculated Nt with hot KCl extractable N. Our results indicated that the potential mineralizable N released from soil differed among different eco-regions and slope positions. Potential mineralizable N is lower in southern Alberta as compared to central Alberta. The lower slopes released more N over higher slope positions. Our results also showed that Nt released in soil over the growing season correlated with hot KCl extractable N quite well in three different slope positions. However, variability of Nt in upper slopes is greater than that in middle and lower slopes due to a shallow A horizon and variable soil moisture during the growing season. After removal of outliers (9% of the total data set), the values of R2 (regression of hot KCl N with calculated Nt) are 0.529, 0.576 and 0.627 for upper, middle and lower slope position, respectively. Based on our calculated results, a potential mineralizable N map in Alberta can be developed, which will guide producers to manage soil as well as fertilizer N.
Introduction
In Alberta, nitrogen fertilizer recommendation is based on soil test NO3-N. As early as 1974, Carson et al. (1974) concluded from over 200 field experiments in Alberta over a five-year period that soil NO3-N had a very good predictable value for estimating crop yield, and yield increment from N fertilizer application. Since that, soil NO3-N was adopted as an index for estimating plant available N in soil and was used for fertilizer recommendations in most laboratories in Alberta. Based on this, a computer software was developed (Kryzanowski, 1995).
It is also well known that soil NO3-N changes from time to time depending on mineralization/immobilization of soil organic N. The soil test NO3-N is an instant determination at the time of soil sampling. It may change over time. The change of soil NO3-N or NH4-N concentration is primarily dictated by composition of soil organic matter itself (i.e. C:N ratio) and environmental factors such as soil temperatures and moisture regime. Apart from the soil organic matter composition, numerous efforts have been made to quantify the amount of N available in a growing season or in a year.
Early work by Stanford and Smith (1972) described organic N kinetics in soil as Nt =No(1-e-kt), where Nt is cumulative N release at time t, No is potential mineralizable N and k, the rate constant. To further describe organic N mineralization in soil, Molina et al. (1980) and Bonde and Rosswall (1987) used a two component model to depict the potential mineralizable N: Nt=Na(1-e-ht)+Nr(1-e-kt), where Na and Nr represents the labile organic N and slow organic N pool, respectively. Nt is the cumulative N released at time t, and h and k are first-order rate constant associated with the more available and more recalcitrant fractions, respectively. The impact of soil water content on Nt, however, was not fully considered for both equations. To quantitatively estimate the rate constant in either equation as affected by soil moisture content, Myer et al. (1982) used a "y" factor as a fraction of maximum mineralization rate occurring at about -0.01 to -0.03 MPa soil water content. The arrangement of y factor in the equation of Nt =No(1-e-kt) came in two forms. Griffin and Laine (1983) assumed that k was linearly related to the fraction of optimum available water, and hence the model is Nt =No(1-e-kty). Olness (1984) suggested that the model Nt =No(1-e-kt)y is more appropriate to represent the mineralization process in soil. To determine temperature effect of the rate constant, the Arrhenius equation was often used (Stanford et al., 1973, Campbell et al. 1984).
Apart from using models to estimate Nt over the entire growing season, alternative technologies such as incubation (Jenkinson and Ladd, 1981; Nannipieri et al. 1990; Parkinson and Coleman, 1991), chemical extraction (Bremner, 1965; Keeney, 1982), and near infra-red reflectance (NIR) (Meyer, 1989; Chang et al., 2001) have been utilized. The incubation methods always required a duration of time to conduct the test. The chemical methods, on the other hand, were debatable since a good correlation with the data obtained by other reliable means has been inconsistent (Dahnke and Vasey, 1973; Keeney, 1982). Because of that, Bundy and Meisinger (1994) suggested that chemical extraction is not an ideal option to estimate potential mineralizable N. The NIR method, although it is at development stage, provide some hope for a quick, consistent, and reliable estimation of potential mineralizable N in soil.
Hot KCl extractable N was evaluated extensively in the prairie provinces of Canada, and a good correlation was used to estimate fertilizer requirement (Campbell et al., 1997). Upon examining historic data, Karamanos found that potential mineralizable N often consists of 2.6% of total soil organic N in western Canada (Rigas Karamanos, Westco, Calgary Canada, 2000, personal communication).
Canada has a hierarchical system of ecological land classification based upon similar land and climatic characteristics (Ecological Stratification Working Group, 1995). At the ecodistrict level, Alberta has 109 ecodistricts in the agriculture area of the provinces (about 40 million hactar). Soil quality benchmark sites have established in 42 of these ecodistricts in 1998 (Cannon and Leskiw, 1999). In these sites, soil and crop samples are systematically collected and analyzed every year. Our objectives in this study are to further evaluate hot KCl as a valid method for estimating mineralizable N (Nt) in soil on a year basis from soil samples across landscape and diverse regions, and demonstrate spatial variability of mineralizable N in a field and in regional scale.
Materials and Methods
Benchmark sites (42) were selected in 1998 in agricultural area of Alberta (Figure 1- 267 KB PDF). The 42 soil quality benchmark sites are representative of soil-landscape patterns and agronomic practices in Alberta (Cannon and Leskiw, 1999). Each site includes three landform positions (upper, middle and lower) along a catena. All sampling sites were located with a differential global positioning system to permit future sampling at the same sites. Plant samples were taken each year from a 0.5 m2 area, grain yield and total above ground bio mass were determined on an air-dried basis. The protein content of grain samples were determined using NIR following the standard operating procedure (SCDC, 1995). Soil samples of 0-15 and 15-30 cm depth were taken annually in the fall since the establishment of the sites. Soil samples were air-dried and then sent to laboratory for analysis. Analysis of soil samples at site establishment included soil texture, soil nutrient status (available N, P, K etc.), bulk density, salinity, acidity, organic N and C and inorganic C, hot KCl extractable N, and light fraction C.
To estimate the mineralizable N in soil, we used the model proposed by Olness (1984) Nt =No(1-e-kt)y, where Nt is cumulative N mineralized (ug g-1 wk-1), No is the potential mineralizable nitrogen (ug g-1), k is rate constant (wk-1), and t is time, week. We have determined the temperature effect on rate constant, k, and used a value of 2.6% of total organic N as No. As for the y factor, we used three methods to assess it, 1) assign 0.8, 0.5 and 0.2 for optimal, midium and poor soil water content (Campbell et al., 1988), 2) divide the annual precipitation by the crop maximum water use (i.e. 460 mm) in a yearly basis for each site, and 3) divide the annual growing season (May to August) precipitation by the crop maximum water use (i.e. 460 mm) for each site. We also used a rate constant (k) of 0.028 wk-1 at 25oC, a number derived from many experiments in western Canada (Campbell et al., 1988). We validated the calculated Nt by comparing it with hot KCl (35oC) extractable N at the same site. A regression analysis was made to determine the relationship between the calculated Nt and the hot KCl extractable N.
Campbell et al.(1988) defined No as the amount of N extracted at 35oC. Stanford and Smith (1972) used a mathematical approach to extrapolate No from the known Nt and k. We think that the potential mineralizable N is a fraction of total organic N. The fraction in our soil and climatic condition is as high as 2.6%. The hot KCl extractable N, on the other hand, is an estimation of the Nt in a growing season or a year. Therefore, in our research, we used hot KCl as a validation of calculated Nt rather than using it as No of potential mineralizable N in Nt =No(1-e-kt)y.
Results and Discussion
In Alberta, there are five major ecological regions. From south to north, they are, Mixed Grassland, Moist Mixed Grassland, Aspen Parkland, Boreal Transition, and Peace lowland. From south to north, the precipitation increased and mean temperature decreased. The mean temperature for the entire province is 13.2oC and annual precipitation is about 431.4 mm (Table 1). In general, soil in the south has less organic matter content as compared to the soils in the northern Alberta. The difference between low and high soil total N is as high as 18 times (Table 1).
Table 1. Mean Annual Precipitation, Growing Season Air Temperature, Total N and Hot KCl in Alberta (temperature, precipitation on ecodistrict basis, N on sample site basis).
.
| Temperature | Precipitation | Total N in soil | Hot KCl N in soil |
oC | mm | % | mg kg-1 soil |
Minimum | 6.5 | 307.7 | 0.035 | 6.1 |
Maximum | 20.0 | 540.1 | 0.629 | 65.5 |
Mean | 13.2 | 431.4 | 0.240 | 32.1 |
As it is showed in the Table 1, the disparity between mean minimum and maximum temperature is only 13.5oC. We used two empirical equations developed from Arrhenius function to determine the rate constant k as affected by temperatures, one from Stanford (1973), and the other from Campbell (1984). The calculated k using the function from Stanford et al. (1973) is quite similar among the different temperatures. The maximum value is 0.01547 while the minimum is 0.01222, a difference of only 0.00325 (Table 2). The Arrhenius function of Campbell et al. (1984) yielded small values for k, smaller than most values found in the literature. Given the factor that temperature difference is narrow in our benchmark sites, instead of calculating the "true k" for each daily temperature, we used a constant rate for k of 0.028 week-1 reported by Campbell et al. (1988).
Table 2. Rate Constant Change as a Function of Temperature.
. | Rate constant, k1 | Rate constant, k2 |
Min | 0.01222 | 6.65 10-5 |
Max | 0.01547 | 1.6 10-4 |
Mean | 0.01347 | 9.9 10-5 |
1 log k = 6.16-2299/T, Stanford et al. (1973).
2 log k = 27.33-8973/T, Campbell et al. (1984).
Using this rate constant k and 2.6% of soil organic N as potential No, we calculated the mineralizable N, Nt, for different y values (Nt =No(1-e-kt)y ). The Nt derived from different y values showed that differences exist among the three calculation methods (Table 3). The lowest mean Nt was found with Method 3 and the highest Method 2. Using hot KCl as a relative reference, it showed that Method 2 is a better method as compared to the other two calculation methods. This is also reflected by the regressions of the three methods against hot KCl (35oC ) extractable N (Figure 2). The R2 value of the Method 2 is 0.63, higher than 0.58 for Method 1 and Method 3.
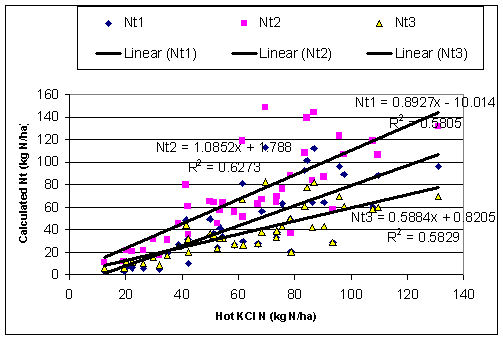
Figure 2. Regressions of three calculated Nt with hot KCl extractable N.
Table 3. Comparison of Nt from Different Values of Moisture Factor, y Using Soils from
Lower Slope Position.
.
| Method 1* | Deviation from Hot KCl N | Method 2 | Deviation from Hot KCl N | Method 3 | Deviation from Hot KCl N |
. | . | kg/ha | . | . | . |
Min. | 2.79 | 9.48 | 5.22 | 7.04 | 2.87 | 9.39 |
Max. | 290.73 | 159.67 | 412.90 | 281.89 | 234.54 | 103.48 |
Mean | 50.16 | 14.00 | 75.39 | 11.23 | 41.00 | 23.16 |
*Method 1- assign 0.8, 0.5 and 0.2 for optimal, middle and poor soil water content.
Method 2 - calculate y by dividing annual precipitation with maximum water use of crops (460 mm).
Method 3 - calculate y by dividing precipitation of growing season (May to August) with maximum water use of
crops (460 mm).
The calculated Nt from Method 2 was regressed against Hot KCl N for each slope position of entire benchmark sites (Figure 3). The R2 for each landscape position originally was poor. The R2 in Figure 3 was achieved after removal of some outliers (9% of the total data set). Originally, the R2 was 0.095, 0.41 and 0.33 for lower, middle and upper slope positions, respectively. When some of the outliers were removed, the R2 was 0.627, 0.576 and 0.529, for lower, middle and upper slope positions, respectively, and they are statistically significant ( p < 0.001). The number of sampling points removed from lower, middle and upper slope positions were 3,1, and 6 because the disparities are big between Nt and hot KCl extractable N in those data points.
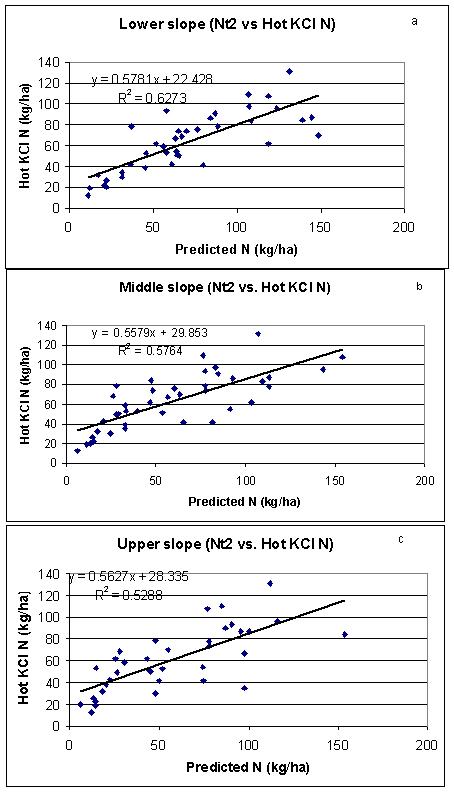
Figure 3. Regression of Nt from (a) lower slope, (b) middle slope and (c) upper slope
positions against hot KCl extractable N.
The spatial distribution of Nt reflects the variance of temperature and moisture along the landscape positions. Mahmoudjafari et al. (1997) reported mineralization rate difference among soils taken from different landscape positions in the field. The average of calculated Nt for all benchmark sites in our research showed that the lower slope positions have higher Nt than middle and upper slope positions (Table 4). Differences in Nt clearly indicated necessity of variable rate of N fertilization. Also the practice of one soil test for one fertilizer recommendation for an entire landscape area can lead inefficient use of N fertilizer. The difference in Nt is much large between upper slope and lower slope positions (23.15 mg N/kg soil or 46.5 kg N/ha), but much less between middle and upper slope positions (6.06 mg N/kg soil or 12.1 kg N/ha). The difference between upper and low slope is about two third of entire year N fertilizer application rate (a 60 kg N/ha is conventionally used in Alberta), large enough to adopt variable rate fertilization practice.
Table 4. Calculated Nt at Different Landscape Positions.
.
| Lower slope | Middle slope | Upper of slope |
mg kg-1 soil | mg kg-1 soil | mg kg-1 soil |
Min | 5.22 | 6.26 | 6.00 |
Max | 412.95 | 154.17 | 153.93 |
Mean | 75.39 | 58.20 | 52.14 |
Comparison of Nt for each ecoregion indicated that Mixed Grassland in the southern Alberta has lower Nt as compared to the northern regions of Peace lowland and Boreal Transition (Table 5). In the Mixed Grassland ecoregion in the southern Alberta, even with high heat unit, less precipitation limited organic N accumulation and mineralization in soil. The mean Nt for lower slope position in the Mixed Grassland ecoregion was about 4 times lower than that found in the Peace lowland ecoregion, and about 6 times lower than the Boreal Transition ecoregion (Table 5). A similar trend was also found for the middle slope position.
Table 5. Calculated Nt at Different Ecoregions at Different Slope Positions.
.
| Peace low land | Boreal transition | Aspen parkland | Moist mixed grass land | Fescue grassland | Mixed grassland |
. | . | mg kg-1 soil | . | . | . |
Lower slope |
Min. | 22.81 | 28.75 | 2.61 | 15.55 | 31.59 | 5.59 |
Max. | 65.73 | 206.48 | 72.15 | 39.93 | 44.13 | 18.11 |
Mean | 38.49 | 65.88 | 34.08 | 25.82 | 37.86 | 10.08 |
Middle slope |
Min. | 16.32 | 12.96 | 5.54 | 12.10 | 28.40 | 3.13 |
Max. | 77.08 | 28.33 | 71.67 | 40.85 | 38.66 | 10.28 |
Mean | 41.15 | 54.75 | 36.07 | 12.88 | 33.53 | 7.04 |
Upper slope |
Min. | 15.16 | 3.63 | 5.95 | 12.80 | 23.85 | 3.00 |
Max. | 55.99 | 27.36 | 58.07 | 48.96 | 48.78 | 11.26 |
Mean | 57.33 | 15.22 | 28.34 | 29.00 | 36.32 | 7.16 |
The amount of mineralizable N between landscape positions within an ecoregion was not as dramatic as the difference among the landscape positions across all benchmark sites (Table 4). The reason for that is sampling population. The total number of samples was 42 for each landscape position for all benchmark sites. However, when samples were grouped into ecoregions, the number of samples per ecoregion reduced substantially. The number of samples was 10, 8, 9, 5, 2, and 7 for Peace lowland, Boreal Transition, Aspen Parkland, Moist Mixed Grassland, Fescue Grassland and Mixed Grassland, respectively. The small number of samples for each ecoregion might not encompass the entire range of Nt distribution along the landscape positions.
In conclusion, hot KCl extractable N is correlated to the calculated mineralizable N, Nt. Mineralizable N varied in landscape positions and ecoregions. This research showed that using a single test of hot KCl can best estimate N released over a year. Thus, a single, realistic soil N test can be developed for nutrient management in crop production. Such a method would help producers to adopt site specific management approach and variable rate fertilization.
Acknowledgement
Western Co-operative Fertilizers Ltd. provided funding for this research. The benchmark sites are supported by the Alberta Environment Sustainable Agriculture (AESA) programs.
Reference
- Bonde, T.A. and T. Rosswall. 1987. Seasonal variation of potentially mineralizable nitrogen in four cropping systems. Soil Sci. Soc. of Amer. J. 51:1508-1514.
- Broersma, K., N.G. Juma and J.A. Robertson. 1996. Net nitrogen mineralization from a Gray Luvisol under diverse cropping systems in the Peace River region of Alberta. Can. J. Soil Sc. 76:117-123.
- Bremner, J.M. 1965. Total nitrogen. pp. 1149-1178. In C.A. Black et al. (eds), Methods of Soil Analysis. Part 2. Chemical and Microbiological Properties. 1st ed. American Society of Agronomy, Madison, WI.
- Bundy, L.G., and .J. Meisiger. 1994. Nitrogen availability indices. pp. 951-984. In: R.W. Weaver et al. (ed.) Methods of Soil Analysis. Part 2. SSSA Book Ser. 5. SSA Madison, WI.
- Campbell, C.A., Y.W. Jame, A. Alil, and J. Schoenau. 1997. Use of hot KCl-NH4-N to estimate fertilizer N requirements. Can. J. Soil Sci. 77:161-166.
- Campbell, C.A., Y.W. Jame, and R. De Jong. 1988. Predicting net mineralization over a growing season: model verification. Can. J. Soil Sci. 68:537-552.
- Campbell, C.A. Y.W. Jame, and G.E. Winkleman. 1984. Mineralisation rate of constants and their use for estimating nitrogen mineralization in some Canadian prairie soils. Can. J. Soil Sc. 64:333-343.
- Cannon, K. and L. Leskiw. 1999. Soil quality benchmarks in Alberta. In Proceedings of the 36th annual Alberta Soil Science Workshop, February 16-18, 1999, Calgary, Alberta.
- Carson, J.A., J.T. Harapiak, A.M.F. Henning, W.E. Janke, M. Nyborg, D.C. Penney, D.R. Walker. 1974. Use of soil testing to predict nitrogen fertilizer needs of barley and rapeseed in Alberta. Proceedings of the Soil Fertility workshop, Feb. Saskatoon, Saskatchewan. pp 74-82.
- Chang, C.W., D. A. Laird, M. J. Mausbach and C.R. Hurburgh Jr. 2001. Near-infrared reflectance spectroscopy-principal components regression analysis of soil properties. 2001. Soil Sci. Soc. Am. J. 65:480-490.
- Dahnke, W.C. and E.H. Vasey. 1973. Testing soils for sitrogen. pp. 97-114. In: L.M. Aalsh and J.D. Beaton (ed.) Soil Testing and Plant Analysis. SSA, Madison, WI.
- Ecological Stratification Working Group. 1995. A National Ecological Framework for Canada. Agriculture and Agri-Food Canada, Research Branch, Centre for Land and Biological Resources Research and Environment Canada, State of the Environment Directorate, Ecozone Analysis Branch, Ottawa/Hull.
- Griffin, G. F. and A.F. Laine. 1983. Nitrogen mineralization in soil previously amended with organic wastes. Agron. J. 75:124-129.
- Keeney, D.R. 1982. Nitrogen availability indices. pp. 711-734. In: A.L. Page et al. (ed.) Methods of soil analysis. Part 2. 2nd ed. ASA and SSSA, Madison, WI.
- Kryzanowski, L. 1995 AFFIRM: Alberta farm fertilizer information and recommendation manager. Conservation Workshop, Nov. 26-28, 1995 Saskatoon, Saskatchewan Canada.
- Jenkinson, D.S. and J.N. Ladd. 1981. Microbial biomass in soil: Measurement and turnover. P. 415-417. In E.A. Paul and J.N. Ladd (eds) Soil Biochemistry. Vol. 5. Macel Dekker, Inc. NY.
- Molina, J. A. E., C.E. Clapp and W.E. Larson. 1980. Potentially mineralizable nitrogen in soil: the simple exponential model does not apply for the 23 weeks of incubation. Soil Sci. Soc. Amer. J. 44:442-443.
- Myers, R. J. K., C. A. Campbell and K.L. Weier. 1982. Quantitative relationship between net nitrogen mineralization and moisture content of soils. Can. J. Soil Sci. 62:111-124.
- Meyer, J.H. 1989. Rapid simultaneous rating of soil texture, organic matter, total nitrogen and nitrogen mineralization potential by near infra-red reflectance. S.Afr. J. Plant Soil. 6:59-63.
- Nannipieri, P., S. Grego, and B. Ceccanti. 1990. Ecological significance of the biological activity in soil p 239-355. In J.M. and G. Stotzky (eds) Soil Biochemistry. Vol 6. Marcel Dekker, Inc. NY.
- Olness, A. 1984. Re:Nitrogen mineralization potentials, No and correlations with maize response. Agron. J. 76:171-172.
- Parkison, D., and D.C. Coleman. 1991. Microbial communities, activity and biomass. Agric. Ecosystems Environ. 34:3-33.
- SCDC, 1995. Standard Operating Procedures Manual, Soil and Crop Diagnostic Centre, Alberta Agriculture, Food and Rural Development, Edmonton, Alberta, Canada.
- Stanford, G., M.H. Frere and D.H. Schwaninger. 1973. Temperature coefficient of soil nitrogen mineralization. Soil Sci. 115:311-323.
- Stanford, G. and S.J Smith. 1972. Mitrogen mineralization potentials of soils. Soil Sci. Soc. Amer.Proc. 36:465-472.
Prepared by
Mingchu Zhanga, R. E. Karamanosb, L. M. Kryzanowskia, K. R. Cannonc, and T. W. Goddardc
aAgronomy Unit, Alberta Agriculture, Food and Rural Development, 6903-116 Street, Edmonton, Alberta, Canada T6H 4P2. email:mingchu.zhang@gov.ab.ca
bWestco, Box 2500, Calgary, Alberta, Canada T2P 2N1
cConservation and Development, Alberta Agriculture, Food and Rural Development, 7000-113 Street, Edmonton, Alberta, Canada, T6H 5T6 |
|