| Executive summary | Introduction | Materials and methods | Results | Discussion | Summary and conclusions | References | Acknowledgements
The figures referenced in this paper are available in the full 69 page (4.5MB PDF file) Preliminary Assessment of Available Soil P in Alberta: Status and Trends - It will take you approximately 22 minutes to download if your connection speed is 28.8KB.
Executive summary
Change in soil available phosphorus (P) in Alberta was determined by comparing results for agricultural soils submitted by farmers for routine analysis over two time periods (1963-67 vs. 1993-97). On dryland (non-irrigated) with annual crops, P increased in 15 (24%), decreased in 27 (44%) and did not change in 20 (32%) ecodistricts. Most of the statistically significant increases occurred in southern Alberta. For dryland with perennial crops, P increased in seven (18%), decreased in nine (22%) and did not change in 24 (60%) ecodistricts. For irrigated crops in southern Alberta (nine ecodistricts), there was a statistically significant increase in P for annual crops in four ecodistricts, and for perennial crops in one ecodistrict, with no changes detected in the other ecodistricts.
It appears that available soil P did not increase consistently from the 1960's to the 1990's despite increased fertilizer application rates during the 1950's, 1960's and 1970's, which were generally maintained into the 1980's and 1990's. One possible explanation is that relatively higher nitrogen rates, with concomitant increases in crop yields, has resulted in increased uptake of soil and fertilizer P. Another possibility is the changes in application technology, such as banding, to increase the efficiency of uptake of applied P.
These advancements have generally been applied more extensively to annual than to perennial cropping systems, which may explain why there was overall less of a decrease and more of a nochange in P for perennial crops.
Although there are some ecodistricts with a high proportion of fields with excess or optimum soil P for crop production, most soils in Alberta are deficient or marginal in soil P. For the 1993-97 time period 46 out of 62 (74%) ecodistricts for the dryland annual and 35 out of 40 (87%) for the dryland perennial crops had a soil P concentration equal or lower than 25 ppm. Soil P levels and soil testing is required to develop a nutrient management plan to encourage optimum economic crop production.
Descriptive statistics included in this report should be used to gain a more comprehensive characterization of the ecodistrict in terms of soil available P. Particularly, the range, skewness and kurtosis of the data tabulated in the appendices provide details in regards to the number of extreme values relative to the mean.
Introduction
In recent years there has been increased attention to agricultural practices associated with the application and movement of soil phosphorus. The use of fertilizer has increased almost every year since 1945 (Goettel, 1987). Phosphorus fertilizer is added to the soil to provide an adequate supply of this essential nutrient to the crop. Phosphorus is essential for plant life and it ensures a series of functions ranging from the primary mechanisms for energy transfer to the transfer of genetic traits (Wallingford, 1978). Phosphorus is removed from the soil by plant uptake, erosion and runoff. In addition, different crops remove different amounts of P from the soil. Because agricultural practices across the prairie region have been changing over time, it is timely to assess how the use of fertilizer is reflected to the amount of available P found in soil.
The first objective of this project was to produce a preliminary assessment of soil phosphorus levels through ecodistrict estimates, and to determine the changes that have occurred over time. The second objective was to analyze the changes in terms of geographical distribution and identify areas in Alberta in which P amendments could result in a positive crop response. In particular, our analysis should be used as a basis for future research, while attempting to quantify crop response to P in particular areas of Alberta. It is suggested that the outcomes of this work could be used as a basis for:
- crop modelling exercise to integrate soil, climate and management used to generate a first general assessment of the potential crop response;
- further sampling in those ecodistricts that might have excessive P levels;
- for specific areas and based upon more soil testing, to develop nutrient management plans which encourage optimum economic crop production.
Materials and methods
Although we recognize the importance and complexity of the different P forms found in the soil, the discussion focuses on the form of soil P that is readily available for plant uptake and extractable using common tests employed by laboratories in Alberta. Because of the amount of available data measured from producer-supplied samples and the scale of interest (ecodistrict level), an interactive GIS database was developed. This system provides an effective way to store and analyze large amounts of spatial and tabular data needed for the analysis (Schreier et al., 1999). Fig. 2.1 displays ecoregions and ecodistricts of Alberta.
Available data
The original data set provided by the Agronomy Unit and the Soil and Crop Diagnostic Centre of Alberta Agriculture Food and Rural Development (AAFRD), includes more than 150,000 records, and the data set provided by the Norwest Labs contains about 130,000. Soil phosphorus, nitrogen, potassium and sulfur are the measured nutrient elements. Soil pH is available in both databases, and percentage of organic matter is only available in the database supplied by the Northwest Labs. Original data sets were constructed by digitizing the results of the analysis performed on farmers supplied samples. The samples were brought to the laboratories for the purpose of measuring the soil fertility level and obtaining the fertilizer recommendation. In most cases, each record includes the name of the crop that was previously grown together with legal land location and depth of the sampling. During the extraction of the data for our analysis we selected the readings at depth of 0-15 cm and constructed two cropping classes, annuals and perennials. In order to construct these two crop classes, we identified and excluded the records with missing information regarding the cultivated crop, as well as crop types such as horticultural, trees and shrubs.
Crops such as wheat, barley and canola were grouped in the annual crop class, fallow is also part of this group. Grassland, alfalfa and clover are typical examples of the crops included in the perennial's group. Figure 2.2 shows the distribution of the sampling locations across Alberta. Only the locations on agricultural land were subsequently selected for the production of the mean P values at the ecodistrict level. In some cases the measuring protocol used by Norwest Labs had a maximum threshold value of 60 ppm, meaning that no effort was made to measure above this concentration. This approach was motivated by the agronomic use of the results; farmers were usually interested in knowing the range of P in the soil at which the crop would generally respond to P amendment (e.g., about 25-30 ppm). In many other instances, however, no specific maximum value was adopted during the measuring procedure. During our analysis, we investigated the extent to which the maximum threshold of 60 ppm occurred within each ecodistrict. In some ecodistricts only 2-5% of the observations would have values equal to 60 ppm signifying that a maximum threshold was indeed adopted. In some other and fewer cases however, the number of samples that could have tested higher than 60 ppm was between 13-21% of the total data points collected in the ecodistricts. Data provided by the Soil and Crop Diagnostic Centre of Alberta Agriculture Food and Rural Development (AAFRD) had a higher maximum threshold value than the one adopted by Norwest Labs, values as high as 100 ppm were found in the original data set.
Reconciling of the P test methods
We selected two five-year time periods 1963-67 and 1993-97 for comparison. Data recorded over the 1963-67 time period were obtained using the Miller and Axley method (Miller and Axley, 1956), while the data recorded during the 1993-97 time period were obtained using the Norwest modified Kelowna method (Ashworth and Mrazek, 1989). In order to reconcile the discrepancy in the measuring protocol we performed a simple regression analysis (SAS, 1990) using the data collected by McKenzie et al. (1995). In this study soil available P was measured for the same soil sample using a series of different extraction methods (McKenzie et al., 1995). For our analysis we selected the measurements obtained using the Miller and Axley and the Norwest modified Kelowna methods. Because of the pH effect upon availability and extractability of P the data were stratified by three pH classes, acidic (<6.0), neutral (³ 6.0 and < 7.5) and alkaline (>7.5). The intercept, slope and R2 listed in Table 2.2 were used to adjust the measurements obtained using the Miller and Axley method to the Norwest modified Kelowna method. Student's t test was used to evaluate the difference between the ecodistrict means (SAS, 1990). Displaying of this analysis, and of the calculated mean P concentration per ecodistrict, was achieved using ArcView GIS 3.2 (ESRI, 1996).
Table 2.2. Parameters of the Regression Analysis Used to Adjust the Miller and Axley Method to the Norwest Modified Kelowna Method (original data from McKenzie et al., 1995).
 | pH<6.0 | pH (¡Ý 6.0 and < 7.5) | pH >7.5 |
Intercept | 2.42 | 1.79 | 0.88 |
Slope | 0.81 | 0.96 | 4.87 |
R2 | 0.88 | 0.89 | 0.66 |
N | 81 | 169 | 18 |
Results
Descriptive statistics
In this analysis, we report some summary statistics that should facilitate the characterization of the data at the ecodistrict level. Each table in the attached appendix contains the number of observations used to calculate the mean representative value, and the tabulated minimum and maximum values to further characterize the information given by the range. Spreading of the values around the mean is described by the standard deviation (s). A measure of the shape of the data set is captured by the coefficient of skewness (Skew), in particular the symmetry of the data is described by the sign. A positive sign of the skewness indicates a longtail of high values to the right making the median less than the mean. If the data have a tail ofvalues to the left, then the coefficient of skewness is negative, making the median greater than the mean. If the skewness is close to zero, this indicates that the histogram is probably symmetric and the median is close to the mean. Another parameter that describes the shape of the data is thecoefficient of variation (CV), which is the ratio of the standard deviation to the mean multiplied by 100. The coefficient of variation can be used to quickly identify some potential problems. In particular, a coefficient of variation greater than 100 is due to the presence of some high values in the sample that may have an effect on the final estimates of the mean. Finally, the kurtosis(Kurt) quantifies the peakedness or flatness of the distribution as compared to the normal distribution. A positive value indicates a relatively peaked distribution and a negative value a relatively flat one. Large values of skewness and kurtosis, however, should be carefully considered since they may indicate that statistical methods based on normality assumptions maybe inappropriate.
Measured values were used to compute the mean value for the ecodistrict and the descriptive statistics regarding the spread and shape of the data. The analysis of the soil availableP was grouped in two time periods (1963-67 and 1993-97) and changes that occurred over time were also considered. In an attempt to characterize and explain the findings of soil P, the soil pHand organic matter content were also analyzed for the 1993-97 time period. Soil pH is animportant parameter to be considered since it relates to the availability and extractability of soilP. Soil organic matter content was also considered since it is an important state variable that connects to the total soil P cycle (Gressel and McColl, 1997).The following paragraphs give details in regards to the soil available P mean for dryland annual and perennial crops, irrigated annual and perennials.
Soil P for dryland annual crops
Table 3.1.1. Summary Table of the Changes in Available Soil Phosphorus in the 0-0.15 m Soil Profile for Dryland Annual Crops (1963-67 vs. 1993-97).
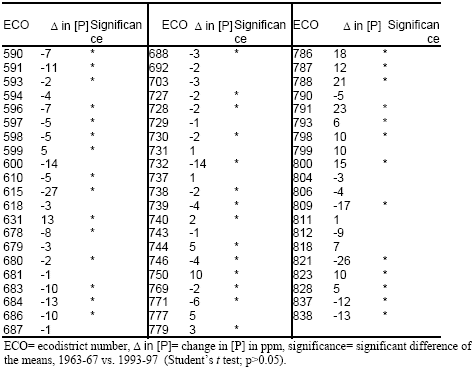
Soil P for irrigated annual crops
Summary statistics for the observations collected in nine ecodistricts during the 1963-67 and the 1993-97 time period are tabulated in Appendices 3.1.2 and 3.1.6. As in the case of dryland annual crops the coefficient of variation is generally higher in the 1963-67 time period, indicating more frequent occurrence of high values in the soil available P concentration.
Likewise, the ranges of values are also higher in the data set collected during 1963-67, reflecting higher maximum values. Slightly higher standard deviation is found in the set of measurements collected in the 60’s. Skewness of the older data set is greater than the one of the data set collected in the 90's. Therefore, the histogram of the latter is probably more symmetric than the former one. Kurtosis of the data set collected in the 90’s has a relatively high number of negative values representing a probable flat distribution of the observed values. Kurtosis of the data collected in the 60’s is always positive indicating a probable peaked distribution compared to normal.
Increase in soil available P from the 60’s to the 90’s tested statistically significant in three ecodistricts and remained unchanged in the other six (Table 3.1.2).
Table 3.1.2. Summary Table of the Changes in Available Soil Phosphorus in the 0-0.15 m Soil Profile for Irrigated Annual Crops (1963-67 vs. 1993-97).
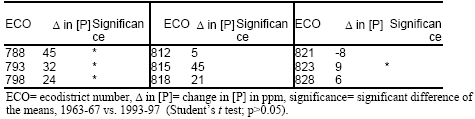
Soil P for dryland perennial crops
Appendices 3.1.3 and 3.1.7 contain the summary statistics of soil available P for dryland perennial crops for the 1963-67 and for the 1993-97 time periods. As in the previous cases, the coefficient of variation is generally higher in the 1963-67 time period indicating more frequent occurrence of high values in the soil available P concentration. The ranges of values are also higher in the data set collected during 1963-67 reflecting higher maximum values. Standard deviation and skewness for the data of the 60’s are also higher than the ones of the 90’s data indicating that 60’s data set might have more variability in the observed values. Variability of the measured values is also corroborated by the higher CV found in the older data set. Kurtosis is also higher for the data collected in the 60’s, indicating that the distribution of the data is relatively more peaked.
Soil available P from the 60’s compared to the 90’s remained unchanged in 24, increased in seven, and decreased in nine ecodistricts (Table 3.1.3).
Table 3.1.3. Summary Table of the Changes in Available Soil Phosphorus in the 0-0.15 m Soil Profile for Dryland Perennial Crops (1963-67 vs. 1993-97).
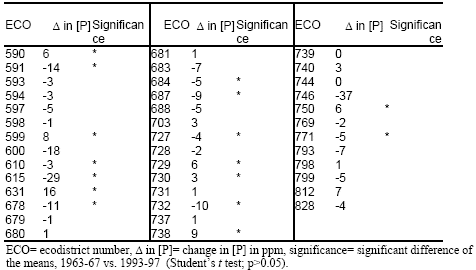
Soil P for irrigated perennial crops
Summary statistics of the soil available P for irrigated perennial crops (seven ecodistricts) for the 1963-67 and for the 1993-97 time periods are tabulated in Appendices 3.1.4 and 3.1.8, respectively. Coefficient of variation is generally higher for the 1963-67 time period, indicating frequent occurrence of high values in the soil available P concentration. The ranges of values are also higher in the data set collected during 1963-67, reflecting higher maximum values. The same trend is observed for the standard deviation, which is generally higher in the 60’s data set. Insight with regards of the shape of the histogram can be gained by examining the skewness of the data. For the 1963-67 time period all the ecodistricts have a positive skewness signifying that the data set is composed by some high values positioned on the right portion of the histogram. Data collected during the 1993-97 time period have few skewness values close to zero, indicating symmetry of the histogram. Nevertheless, this data set contains some negative values probably due to some low numbers on the left portion of the histogram. Finally, the data set collected in the 60’s has some relatively higher kurtosis values than the 90’s data set, signifying a more peaked distribution of the data.
Increase in soil available P between the 1963-67 and 1993-97 time period was statistically significant in only one ecodistrict. Non-significant changes were detected in all the other ecodistricts (Table 3.1.4).
Table 3.1.4. Summary Table of the Changes in Available Soil Phosphorus in the 0-0.15 m Soil Profile for Irrigated Perennial Crops (1963-67 vs. 1993-97).
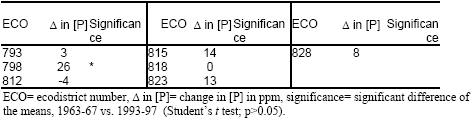
Soil pH for dryland annual crops
Further characterization of the current soil available P was considered by analyzing the soil pH values per ecodistrict with particular emphasis on how soil pH affects availability and extractability of soil P.
Plant uptake of P is largely in the form of orthophosphate ions (H2PO4 - and HPO4 2-). Each form is present in the soil in different amounts depending on the soil pH level. At pH of 7.2 the amount of the two orthophosphates is about equal, below pH 7.2 primary orthophosphate (H2PO4 -) accounts for the majority of P available to plants. With pH above 7.2 the majority of P is in the form of secondary orthophosphate (HPO4 2-). Uptake of this ion by plants is slower than for the primary orthophosphate ion (Mulla and Schepers, 1997).
For the dryland annual crops the pH mean values, with some summary statistics, are reported in Appendix 3.1.9. Coefficients of variation are low indicating a low variability in the measured data set. The relatively contained spread of the measurements is also indicated by the low standard deviations. Minimum and maximum values of the observations produce a range that is usually close to two or three pH units. Skewness is usually close to zero indicating a probable symmetry of the histogram characterized by the median positioned close to the mean. Kurtosis values are mostly negative pointing out that these data may have a relative flat distribution as compared to the normal distribution.
Soil pH for irrigated annual crops
Appendix 3.1.10 reports mean soil pH values with the summary statistics for the irrigated annual crops. The spread of the data is similar to that of the dryland annual crops. Skewness values tend to be negative but always close to zero. Therefore, a relative symmetry of the histogram is expected. Kurtosis is also negative in most ecodistricts indicating a relatively more flat distribution than the normal.
Soil pH for dryland perennial crops
In Appendix 3.1.11 the mean soil pH values are listed together with the relative summary statistics. In terms of spread, these data have some similarity with the other pH measurements previously discussed. Skewness values tend to be low and usually positive indicating some degree of symmetry in the histogram. Kurtosis values are also relatively low with about an equal occurrence of negative and positive values.
Soil pH for irrigated perennial crops
Soil pH statistics for the irrigated perennial crops is characterized by a low spreading of the data. This is confirmed by low standard deviation and by a small coefficient of variation that never exceeds 11%, and the values of the range also being small (Appendix 3.1.12). Skewness of the data is usually around zero, with Kurtosis values that tend to be negative indicating some degree of flatness in the distribution of the observations.
Soil OM for dryland annual crops
Ecodistrict estimates of soil organic matter for dryland crops are tabulated on Appendix 3.1.13. An interpretation of the descriptive statistics reveals that the coefficient of variation is more than 100 on a few occasions. High maximum values in the data set caused the range to reach some high values (e.g., about 38). Standard deviation is usually around two. Skewness of the data is usually small, with positive values indicating the presence of some high numbers on the right of the mean. In some cases, the distribution of the data with respect to normal is
probably highly peaked due to some positive kurtosis values.
Soil OM for irrigated annual crops
For the irrigated annual crops, the soil organic matter content has a coefficient of variation that is below 25 in most ecodistricts, and exceeds 100 only in one ecodistrict (Appendix 3.1.14). Standard deviation is usually below 0.5, and values of the range are usually small and only in one occasion equal to 21. Skewness of the data is usually small indicating some degree of symmetry in the histogram of the data. Kurtosis values are usually negative indicating that the distribution of the data may be more flat than the normal distribution.
Soil OM for dryland perennial crops
Descriptive statistics of the soil organic matter content for dryland perennial crops is summarized in Appendix 3.1.15. High values of the coefficient of variation are rare, and usually corresponding to high values associated with a relatively higher standard deviation. High range values (e.g., about 30) are usually associated with the high maximum value in the observations. Skewness is usually close to one or zero indicating some degree of symmetry. High skewness values reflect the presence of a high value in the set of measurements. Kurtosis ranges from negative to high positive values indicating some degree of flatness in the distribution in the first case, and some probable extremely peaked distribution in the second case.
Soil OM for irrigated perennial crops
Irrigated perennial crops have a fairly low coefficient of variation that is partially reflected in the low standard deviation (Appendix 3.1.16). Values of the range are also small and paralleled by low maximum values. Skewness is around zero for the majority of the ecodistricts indicating some symmetry of the histogram. Kurtosis is mainly negative representing a distribution flatter than the normal.
Spatial distribution
The results of the statistical analysis discussed in the previous sections were used to create a series of interactive GIS (Geographical Information System) databases. This approach provides an effective way to store and analyze large amounts of spatial and tabular data needed for the spatial analysis (Schreier et al., 1999). A series of maps has been produced for each time period (1963-67 and 1993-97), and each cropping scenario (dryland annual and perennial; irrigated annual and perennial). Displaying of the mean available P for the ecodistrict was achieved by generating four classes while taking into account the natural breaks occurring in the data sets. Changes in soil available P that occurred from the 60’s to the 90’s were displayed in a different set of maps which synthesized the absolute change in soil available P, and the statistical significance of the changes. Student’s t test was used to examine the statistical significance of two means from uneven sample size.
In the attempt to produce a more comprehensive analysis we produced a series of maps that summarized the mean soil pH and organic matter for the 1993-97 time period. Soil pH was regarded as an important parameter for the discussion since it ties with the plant uptake of P. Plants uptake phosphorus largely in the form of orthophosphate ions (H2PO4 - and HPO4 2-). Each form is present in the soil in different amounts depending on the pH level. At pH of 7.2 the amount of the two orthophosphates is about equal, below 7.2 H2PO4 - accounts for the majority of the orthophosphates available to plants. With pH above 7.2 the majority of the orthophosphates are in the form of HPO4 2-, uptake of the latter by plant is slower than for the H2PO4 - orthophosphate ion (Tisdale et al., 1993). Organic matter found in the soil has a net effect on the total phosphorus in the soil because of the potential role in releasing or immobilizing it. Presence of organic compounds due to the decomposition of organic residues seems to increase the availability of P (Tisdale et al., 1993). Major processes for availability of P from organic matter are: a) formation of organophosphate compounds available for plant assimilation; b) anion replacement of H2PO4 - on adsorption soil sites.
Mapping of the dryland annual crops scenario
In Figure 3.1 the mean available soil P is shown for each ecodistrict during the 1963-67 time period and for the dryland annual crops. The majority of the ecodistricts (i.e., 27) have a soil P concentration of 20-25 ppm, 19 ecodistricts 26-34 ppm, 13 ecodistricts 11-19 ppm and only three ecodistricts 35-45 ppm. For the 1993-97 time period 25 ecodistricts have a soil P level of 20-25 ppm, 20 ecodistricts 12-19 ppm, 12 ecodistricts 26-34 ppm, and five ecodistricts 35-47 ppm (Fig. 3.2). A comparison of the two time periods (1963-67 vs. 1993-97) indicates that soil available P for dryland annual crops did not change in 20 ecodistricts, increased in 15, and decreased in 27. The majority of the ecodistricts with the highest statistically significant increase in soil P are located in the southern part of Alberta (Fig. 3.3).
An attempt was also made to relate our mapping of soil P to physical patterns of the landforms. The changes in soil P that occurred with time were overlaid with the ecoregions compiled by the Ecological Stratification Working Group (1995). Compilation of ecoregions took into account distinctive large order landforms or groups of the latter, or in some cases smaller regional landforms and combined with macro or mesoclimates, vegetation, soil and water patterns. The largest clustering of the increase in soil P is located in the Moist Mixed Grassland and extends northward in the Aspen Parkland, eastward in the Mixed Grassland and westward in the Fescue Grasslands (Fig.3.13). These spatial patterns overlay a series of different soil groups such as the Brown, Dark Brown and Black Chernozemics.
However, our results do not show a strong spatial correlation that is consistently explainable by physical patterns of the landforms grouped in the ecoregions. This leads to the possibility that changes in soil P were largely due to anthropogenic activities. Mapping of the soil pH for the 1993-97 time period shows that the majority of the ecodistricts have a mean pH level below or equal to 7.1 while the higher values (equal or greater than 7.2) are clustered in the southern part of the province (Fig. 3.15). Potential availability of HPO4 - orthophosphate ion for plant uptake is therefore dominant in most parts of the province. The southern part of Alberta is characterized by a potential higher availability of the HPO4 2- orthophosphate ion which is taken up by plants at a relatively slower rate. This situation could play an important role in terms of available P for plant uptake and therefore to the amount of soil P residing in the soil. As previously indicated, another soil property that could play a role in the availability of soil P is the fraction of soil organic matter. The majority of the ecodistricts with lowest values of organic matter (2.1 – 3.8 %) are found in the southern part of Alberta (Fig. 3.19).
Mapping of the irrigated annual crops scenario
Ecodistricts with irrigated annual crops are located in the southern part of Alberta, for this analysis we were able to use a total of nine ecodistricts. For the 1963-67 time period, five ecodistricts had a P level within the 34-40 ppm range, two ecodistricts with 26-28 ppm, one ecodistrict with 29-33 ppm, and one ecodistrict with 41-57 ppm (Fig 3.4). During the later period (1993-97) three ecodistricts had a P level within the 30-38 ppm range, three ecodistricts 58-82 ppm, two ecodistricts 39-50 ppm, and one ecodistrict 51-57 ppm (Fig. 3.5). Changes that occurred over time (1963-67 vs. 1993-97) were non-significant for six ecodistricts while the other three ecodistricts had an increase that was statistically significant (Fig. 3.6). The significant increase in P level was actually in the 11-32 ppm range for two ecodistricts and in the 1-10 ppm range for the other ecodistrict. Mean soil pH level is mainly in the range above or equal to 7.2 (Fig. 3.16) creating the conditions for the orthophosphate to be mainly available in the form of
HPO4 2- ions which is the form that is taken up at a slower rate by plants. Most ecodistricts have a mean soil organic matter level between 2.5 and 2.8% (Fig. 3.20).
Mapping of the dryland perennial crops scenario
Dryland perennials are cultivated in ecodistricts that are distributed across the agricultural areas of Alberta. For the 1963-67 time period, 17 ecodistricts had mean available P values within the 16-21 ppm range, 11 ecodistricts with 22-32 ppm, eight ecodistricts with 11-15 ppm and four ecodistricts with 33-47 ppm (Fig. 3.7). Analysis of the 1993-97 data resulted in 20 ecodistricts in the 16-21 ppm range, nine ecodistricts with 11-15 ppm, eight ecodistricts with 22-28 ppm, and three ecodistricts with 29-40 ppm (Fig. 3.8). Soil available P for dryland perennial crops did not change in 24 ecodistricts, increased in seven and decreased in nine (Fig. 3.9). Increase in P levels that were statistically significant are not clustered around a specific regional area and the available soil P in these ecodistricts increased by about 2-16 ppm. Ecodistricts with unchanged levels in soil P are found over a wide range of ecoregions spanning from the Mixed and Moist Mixed Grasslands to the Boreal Transition (Fig. 3.14). As for the dryland annual crops, the soil pH level is below or equal to 7.1 in the majority of the ecodistricts; higher pH values are clustered in the southern part of the province (Fig. 3.17). If we extrapolate these findings to the availability of orthophosphate ions, we can speculate that plant uptake of orthophosphate could happen at a higher rate for the majority of ecodistricts. Ecodistricts with the lowest mean organic matter (2.3 – 4.4%) are scattered across the province, while ecodistricts with highest organic matter levels (6.8-10.1%) are found in the central-western part of Alberta (Fig. 3.21).
Mapping of the irrigated perennial crops scenario
As in the case of the irrigated annual crops the irrigated perennials are found in the ecodistricts located in the southern part of Alberta. The 1963-67 data set is composed of three ecodistricts with a P level in the 32-40 ppm range, two ecodistricts with 23-26 ppm, one ecodistrict with 27-31 ppm, and one ecodistrict with 41-46 ppm (Fig. 3.10). During the 1993-97 time period two ecodistricts had a P level of 27-40 ppm, two ecodistricts 41-49 ppm, two ecodistricts 50-53 ppm, and one ecodistrict 26 ppm (Fig. 3.11). A statistically significant increase in P level was observed in only one ecodistrict with an increment of 27 ppm (Fig. 3.12). As in the case of irrigated annual crops the soil pH level is equal to or above 7.3 creating the conditions for orthophosphate availability in the HPO4 2- ion form which is taken up by plants at relative slower rate (Fig. 3.18). Soil organic matter ranges between 2.0 and 5.1%, with four out of nine ecodistricts having a mean soil organic matter content of 2-2.5%.
Discussion
Comparison of the two time periods (1963-67 vs. 1993-97) highlighted a relatively higher level of variability in the older data set. Descriptive statistics were used to characterize the available P data. Analysis of spread and shape of the observations indicates that more extreme values are found in the data collected in the 1963-67 time period. The methodology is based on the use of two different data sets constructed by two different entities. Therefore, the results of the descriptive statistics could be used to analyze some of the potential or intrinsic problems of our approach. In some cases, the measuring protocol of available P used by Norwest Labs had a preset maximum threshold value of 60 ppm meaning that no effort was made to measure above this preset concentration. Norwest’s approach is justifiable whenever the analysis of the results is used to formulate fertilizer recommendations, but it does not offer any details regarding sites with excessive amounts of available P. During our analysis we investigated up to which extent the maximum threshold of 60 ppm occurred in the data. Usually, only 2-5% of the observations collected within an ecodistrict would have values equal to 60 ppm indicating that a maximum threshold was indeed adopted. In some other and fewer ecodistricts the number of observations that could have tested higher than 60 ppm is about 13-21% of the total data points in the ecodistricts. On the other hand, the data provided by the Soil and Crop Diagnostic Centre of the Alberta Agriculture Food and Rural Development (AAFRD) had a higher maximum threshold value than the one used by the Norwest Labs; values as high as 100 ppm were found in the original data set. Our analysis is based on voluntary sampling, meaning that the samples were brought in by farmers and were not collected according to a statistical design for the whole province. Therefore, we cannot determine the exact causes for the higher spreading in the data collected in the 60’s.
Despite these uncertainties, the two existing databases allowed the implementation on an analysis based upon a large number of observations, and in fact carries the intrinsic advantage of more uniform spatial coverage across the province. Descriptive statistics in the Appendices could be used to determine the level of confidence we should apply while interpreting the mean soil P for the ecodistrict. Each ecodistrict we analyzed has a line of descriptive statistics that includes information about the shape and spread of the observations. This preliminary assessment of soil P in Alberta could also be used as a base for further investigations. For example, an ecodistrict that in the 60’s had a pronounced skewness value, which subsequently dropped in the 90’s could indicate that over time the occurrence of extreme values, higher or lower than the mean has dropped. However, in order to substantiate this assumption more sampling may be needed; therefore if a newly designed sampling protocol produces data with a low skewness this may indicate that the mean and mode are close together. Therefore, the low occurrence of extreme values would corroborate our initial assumption and could perhaps be used as an analog in other ecodistricts.
Alternatively, we could speculate that whenever the maximum value for the ecodistrict is found to be equal to 60 ppm for the 90’s data, chances are that some soil samples might test higher in available P. Therefore, some more sampling within the ecodistrict might be useful in determining the occurrence of areas with a soil P value well above the typical agronomic level (e.g., about 25-30 ppm).
Since a relatively small portion of observations (e.g., 2-5 % and 13-21% depending on the ecodistrict) reached the preset value of 60 ppm imposed in some cases by Norwest Labs, we can retain a good level of confidence in our estimates of soil P mean. Descriptive statistics in Appendices 3.1.1 through 3.1.8 could be used to identify those ecodistricts in which the maximum value is equal to 60 ppm suggesting that some spots within the ecodistricts have soil P values that exceed 60 ppm. This information becomes useful when designing a field sampling program, which has the objective of identifying areas with excessive amounts of P in the soil. For the 1993-97 time period, 27 out 62 ecodistricts for the dryland annual, 15 out of 40 for the dryland perennial, one out nine ecodistricts for the irrigated annual, and none for the irrigated perennials has data in which the maximum observed value equals 60 ppm.
Temporal and spatial patterns
One of our objectives was first to investigate some of the changes in soil P that took place over time and secondly to analyze the changes in terms of geographical distribution across the province. A comparison of the two time periods (1963-67 vs. 1993-97) indicates that soil available P for dryland annual crops did not change in 20 ecodistricts, increased in 15, and decreased in 27 (Fig. 3.3). The majority of the significant increase in soil P occurred in the southern part of Alberta (Fig. 3.3). Plant available soil P for dryland perennial crops did not change in 24 ecodistricts, increased in seven, and decreased in nine, the unchanged levels in soil P are found across the province (Fig. 3.9).
Irrigated crops are mainly found in southern Alberta. Over time, increases in soil available P for the irrigated annual crops tested statistically significant in three ecodistricts and remained unchanged in the other six (Fig. 3.6). During the same time period, irrigated perennial crops had an increase in soil available P that was statistically significant only in one ecodistrict. Non-significant changes were detected in all the other ecodistricts (Fig. 3.12).
Historically, the use of commercial fertilizer in both the U.S. and Canada increased during the 1950’s, 1960’s and 1970’s primarily by higher application rates. An analysis of the data provided by Agriculture and Agri-Food Canada (Korol and Rattray, 1999) reveals that the phosphate sales in Alberta have increased at a lower rate than nitrogen sales. In particular, phosphate sales doubled from 1968 to 1998 while nitrogen sales increased five times over the same period. These data represent the volume sold by retailers within Alberta and do not include inter-provincial purchases of fertilizers. Therefore, these figures may not correspond exactly to the whole consumption (Korol and Rattray, 1999). In the attempt to link the changes in plant available soil P and the trend in fertilizer sales, a more a complex data analysis should be carried out.
Although a number of cooccurring factors should be considered, it is possible that higher use of N fertilizer results in a higher biomass per m2. Therefore, if the amount of applied P fertilizer is not proportionally increased, the net uptake of P per volume of soil increases because of the higher amount biomass. This may result in a decrease in plant available soil P.
An increase in yields for the annual crops, from the 60’s to the 90’s is captured in Fig. 4.1. An increase in perennial yields is also found, but yield data for the late 90’s are characterized by a decreasing trend. Annual crop values were obtained by averaging the yields of wheat, oats, barley, rye, canola and flax; perennials include only tame hay. Therefore, these data should be considered as only indicative for Alberta since they do not consider all the annual and perennial crops used in the analysis of soil P.
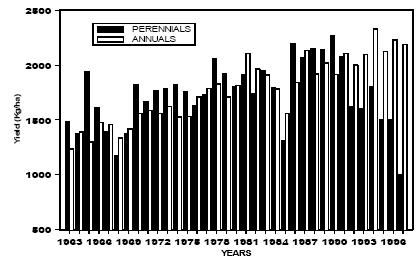
Fig. 4.1. Provincial yield averages grouped by annual and perennial crop classes. Original source of the data: Agriculture Division, Statistics Canada.
At first the changes that took place over time were more numerous on those soils cultivated with annual crops than on those cultivated with perennials. One of the possible reasons for having the soils cultivated with perennial crops being less affected by changes in soil P over time could be related to changes in management practices. Although a specific investigation is needed, we can hypothesize that changes in the technology that could affect the rational use of fertilizer had been more widely adopted in the production of annual crops. Phosphate ions tend to react with soils and become less mobile via sorption, precipitation and chelation. In acid soil, iron and aluminum tend to immobilize phosphate, whereas, in alkaline soil calcium does the immobilization. Fertilizer banded applications could trigger a more economical use of P by placing the fertilizer closer to the roots. Traditionally, the phosphorus fertilizer has been applied in-rows for cereal crops and top dressed for forage crops (Robertson, 1982). During the 80’s the success in deep banding of nitrogen fertilizer stimulated the introduction of new application techniques for phosphorus. Good response obtained with banded or seed placed application of P fertilizer to annual crops has been the triggering factor in changing fertilizer practices toward a more economical use of P.
Although application of P fertilizer can improve dry matter yields and extend the growing season of perennials (Mahli, 1993), application of phosphorus fertilizers is still mainly restricted to top-dressing. Banded application below the surface of established perennial stands could cause serious damage to the roots, and therefore impair P uptake.
Major technological advancements in the way P fertilizer are applied to perennial crops have not been reported. Therefore, we could speculate that the lack of major changes in technology could be the cause for the higher number of ecodistricts that show no changes in the soil P level over time.
In an attempt to link the changes of soil P to land physical properties we found that the largest clustering of the increase in soil P occurred in the Moist Mixed Grassland extending northward in the Aspen Parkland, eastward in the Mixed Grassland and westward in the Fescue Grasslands (Fig.3.13). These spatial patterns overlay with a series of different soil groups such as the Brown, Dark Brown and Black Chernozemics. Increase in P levels that were statistically significant are not clustered around a specific regional area, with the available soil P in these ecodistricts having increased by about 2-16 ppm. For the dryland perennial crops we identified ecodistricts with a non-significant change in soil P over a wide range of ecoregions, spanning from the Mixed and Moist Mixed Grasslands to the Boreal Transition (Fig. 3.14). However, our findings did not reveal a strong spatial correlation that is consistently explainable by physical patterns of the landforms (i.e., ecoregions), leading to the possibility that changes in soil P were largely due to anthropogenic activities.
Soil available P levels and potential crop response to fertilizer
Assessment of soil available P has been the objective of previous studies. For example the Potash & Phosphate Institute (PPI) produced a report on soil samples across the United States and part of Canada, and indicated that 65% of the soil samples collected in Alberta tested medium or lower in soil P level (PPI, 1998). Soil testing medium and low in soil P are those in which an additional P amendment generally results in a crop yield response. In our investigation, we probably used a higher number of observations and refined our analysis at a more detailed spatial scale (i.e., ecodistrict). Our conclusions are similar to those released by PPI. For the 1993-97 time period 46 out of 62 ecodistricts for the dryland annual, and 35 out of 40 for the dryland perennial crops had a soil P concentration equal or lower than 25 ppm. We found that one out of nine ecodistricts for irrigated annual crops, and one out of eight for the irrigated perennial crops, have a mean soil P value between 25 and 30 ppm.
This macro scale scenario for Alberta corroborates with previous findings (PPI, 1998) but with improved precision and more refined spatial scale. Our findings, however, cannot be used to directly infer future yields as a function of increased fertilization rates in those ecodistricts with soil P value between 25 and 30 ppm. Instead, a more detailed analysis with the objective of verifying the potential for crop response at the field level should be implemented. For example we suggest that the current analysis could be combined with a crop modelling exercise to include the potential effects of soil type and climate that are found in particular ecodistricts. Although model simulations provide results that resemble and not necessarily reproduce exactly the full field conditions, this approach may provide preliminary information before the actual implementation of field trials. Other investigators used this approach to evaluate the general crop response to change in boundary conditions such as climate (Izaurralde et al., 1999; Phillips et al., 1996). Similarly a preliminary regional assessment of the potential increase in crop productivity as a result of more P application could be obtained through the use of similar models. However, our work is meant to support regional assessments and not to replace detailed field-testing procedures used to formulate recommendations that apply at a more detailed scale (e.g., single field). Regardless, our analysis shows that currently there are large areas of Alberta that have low to moderate soil P levels and soil testing is required to develop a nutrient management plan to encourage optimum economic crop production.
Summary and Conclusions
Over the 30-year period the mean soil P for the majority of the ecodistricts remained unchanged or decreased. During the 1993-97 time period the majority of the ecodistricts had a mean soil P value between 25 and 30 ppm. Although our results support the fact that on average much of the agricultural soils in Alberta could benefit from P amendment, we recommend that fertilizer management strategies should be examined on a farm by farm basis. An increase in P fertilization may result in higher crop production, but over use of P amendment can be an environmental hazard. Therefore, if the objective is to maximize crop production while controlling P loss from the soil, then the extra P amendment should be considered together with the landscape and climatic characteristics that would have an impact on the P loss. Accepting that strategy, it becomes even more crucial if an extra supply of P is available due to animal production, and therefore the necessity for recycling of this other form of P becomes more of a priority.
In order to produce a comparison between the data collected in the 60’s and the data collected in the 90’s a regression analysis was performed using the data set of McKenzie et al. (1995). A good correlation between the Norwest soil test method and the Miller and Axley method was found for neutral and acid soils, but as the soil pH increased the correlation worsens, similar conclusions were reached by Ashworth and Mrazek (1989). Calculated intercept and slope values were used to adjust the measurements obtained using the Miller and Axley method to the Norwest modified Kelowna method. However, some of the significant changes that occurred over time in the Southern part of Alberta are found on alkaline soils, and although we accounted for the differences of the two soil tests through a regression analysis some caution should be used in drawing conclusions. Therefore, it is possible that some of the increase in soil P that we see from the 60’s into the 90’s could be in part due to the Miller and Axley unsuitability to extract plant available P in alkaline soils. Ashworth and Mrazek (1989) indicated that the poor correlation found in soils with free lime is presumably due to the sulfuric acid in the Miller and Axley solution being the cause for the extract pH to rise from < 2 to > 6 in some cases. The extract of the other test is well buffered at about pH 4.5.
Because the measuring procedure used in the 60’s was different from the one used in the 90’s, an historical comparison of soil organic matter content was difficult to implement. Therefore, the observations collected in the 90’s were used to map the current scenario across the province. Lowest soil organic matter content (2-5 %), is found in Southern Alberta, whereas the rest of the Province ranges between 6 and 10% depending on crop type and water supply. Soil organic matter was considered because it is a potential source or sink for P, however the rate at which P is released is a function of organic matter decomposition rates. Phosphorus released from organic matter decomposition may be an important fraction of the total soil P. However, reliable estimates could be obtained with a more comprehensive analysis. It is suggested that an attempt to quantify the mineralization rate of P should include incubation experiments as well as considerations of the major factors having an impact on the decomposition rate (e.g., soil pH, soil moisture). However, a previous finding indicates that the mineralization of P is particularly important in natural ecosystems (Polglase et al., 1992) or becomes an important source of plant available P in unfertilized soils (Gressel and McColl 1997).
Finally, it was our objective to provide the reader with descriptive statistics to be used for a more comprehensive characterization of the ecodistrict in terms of soil available P. In particular, the range, skewness and kurtosis of the data, tabulated in the appendices provide details to the number of extreme values relative to the mean.
References
Ashworth, J. and Mrazek, K., 1989. The acetic-fluorite test for available phosphorus and potassium. Proceedings of the 26th Annual Alberta Soil Science Workshop, Feb. 21st-22nd, 1989, pp. 178-182.
Daniel, T.C., Carton, O.T., and Magette, W.L., 1997. Nutrient management planning. In: Phosphorus Loss from Soil to Water, Tunney, H., Carton, O.T., Brookes, P.C., and A.E., Johnston, Eds. CAB International, New York, USA, pp. 297-310.
Ecological Stratification Working Group, 1995. A National Ecological Framework for Canada. 125 pp..
ESRI Inc. 1996. Using ArcView GIS. ESRI Inc. Redlands, CA USA.
Goettel, A.W. 1987. Crop nutrient removal and additions in Alberta. Proceedings of the 24th Alberta Soil Science Workshop, Feb. 17th-18th, 1987, pp. 219-232.
Gressel N., and McColl J.G., 1997. Phosphorus mineralization and organic matter decomposition: a critical review. In: Driven by nature, Cardish, G. and K.E., Giller, Eds. CAB International, London, UK, pp. 297-308.
Izaurralde, R.C., Rosenberg, N.J., Brown, R.A., Legler, D.M., Tiscareño Lòpez, M., and Srinivasan, R., 1999. Modeled effects of moderate and strong ‘Los Niños’ on crop productivity in North America. Agricultural and Forest Meteorology 94: 259-268.
Korol, M., and Rattray, G., 1999. Canadian fertilizer consumption, shipment and trade 1997/1998. Agriculture and Agri-Food Canada, (Internet site: http://www.agr.ca).
Mahli, S.S., Harapiak, J.T., Kryzanowski, L.M. and Ukrainetz, H., 1993. Fertilizer management of forage crops in western Canada. The Proceeding of Western Canada Agronomy Workshop, July 7th-9th, 1993.
McKenzie, R.H., Kryzanowski, L., Cannon, K., Solberg, E., Penney, D., Coy, G., Heaney, D., Harapiak, J. and Flore, N., 1995. Field evaluation of laboratory tests for soil phosphorus. Alberta Agricultural Research Institute, Project No. 90 M230. 36 pp. & XI Appendixes.
Miller, J.R. and Axley, J.H. 1956. Correlation of chemical soil tests for available phosphorus with crop response, including a proposed method. Soil Science 82: 117-127.
Mullar, D.J., and Schepers J.S., 1997. Key processes and properties for site-specific soil and crop management. In: The state of site-specific management for agriculture, ASA-CSSASSSA, Madison, WI, USA, pp. 1-18.
Phillips, D.L., Lee, J.J., and Dodson, R.F., 1996. Sensitivity of the US corn belt to climate change and elevated CO2: I. Corn and soybean yields. Agricultural systems 52: 481-502.
Polglase, P.J., Comerford, N.B. and Jokela, E.J., 1992. Mineralization of nitrogen and phosphorus from soil organic matter in southern pine plantation. Soil Science Society of America 56: 921-927.
PPI/PPIC/FAR, 1998. Soil test levels in North America, summary update. PPI/PPIC/FAR Technical Bulletin 1998-3.
Robertson, J.A., 1982. Fertilizer placement. Alberta Agriculture, Agdex 542-5.
SAS Institute Inc. 1990. SAS user’s guide: Statistics, Version 6 Edition. Cary, NC USA SAS Institute Inc..
Schreier, H., Brown, S., Lavkulich, L.M., Shah, P.B., 1999. Phosphorus dynamics and soil Pfertility constraints in Nepal. Soil Science 164 (5): 341-350.
Tisdale, S. L., Nelson, W.L., Beaton, J.D., and Havlin, J.L., 1993. Soil fertility and fertilizers. Macmillan, New York, USA, pp.176-229.
Wallingford, W. 1978. Phosphorus functions in plants. In: Phosphorus for agriculture, a situation analysis. Potash/Phosphate Institute. 160 pp..
Acknowledgements
We would like to thank Alberta the Environmentally Sustainable Agriculture (AESA) program for providing funding and support, and also Alberta Agriculture Food and Rural Development (AAFRD) Agronomy Unit and Norwest Labs for making the data available. We also gratefully acknowledge Dr. A.M. Johnston, Dr. R. McKenzie, K. Cannon and T. Goddard for their valuable comments and discussion. |
|